Mr Jon White
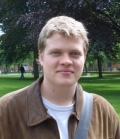
Research Topics:
Small angle neutron scattering from vortex systems
Group:
Small Angle Neutron Scattering
E-mail:
Tel: +44 (0)121 414 4672
Fax: +44 (0) 121 414 4719
Room: East 213
Publications
"Small-angle neutron scattering measurements of the vortex lattice in CaC6"
R. Cubitt, J.S. White, M. Laver et al.
Phys. Rev. B 75, 140516(R) (2007)
DOI: 10.1103/PhysRevB.75.140516
"Superconducting Vortices in CeCoIn5: Toward the Pauli-Limiting Field"
A.D. Bianchi, M. Kenzelmann, L. DeBeer-Schmitt, J.S. White, E.M. Forgan et
al.
Science 319, 177 (2008)
DOI: 10.1126/science.1150600
"Finite gap behaviour in the behaviour in the superconductivity of the
'infinite-layer' n-doped high-Tc superconductor Sr0.9La0.1CuO2"
J.S. White, E.M. Forgan, M. Laver et al.
J. Phys.: Condens. Matter 20, 104237 (2008)
DOI: 10.1088/0953-8984/20/10/104237
I have also been involved with the new results on CeCoIn5. In the Summer 2006 I participated as a Stagiare at the ILL. To read my report on my time there, click here.
Research Overview
My name is Jonathan White and I am currently in my second year of PhD study under the supervision of Professor Ted Forgan in the Condensed Matter Group. My area of research involves the use of the small-angle neutron scattering technique to look at flux line lattice structures in type-II superconductors. My studies are focussed towards improving our fundamental understanding of mainly unconventional superconductors, whose superconducting behaviour is not adequately described by the conventional BCS theory, and that exhibit fundamentally different physics.
Me on the school's annual trip to Coniston
Flux line lattice structures manifest themselves in the mixed state of type-II superconducting materials that are in the presence of moderate applied magnetic fields. In type-II materials, where the interface between a non-superconducting and superconducting material is energetically favoured, (due to the longer extent of the penetration depth over the coherence length) the magnetic field is allowed into the bulk of the superconductor in the formed of quantised flux lines and these flux lines can form a lattice. What sets the flux line lattice apart from a the simple concept of a magnetic field is that flux lines are (in general) actual physically continuous lines of magnetic field, each sustained by a vortex of supercurrent. Magnetic field lines do not actually exist, rather we as the observer superpose these onto our interpretation of the vector field to indicate things such as field direction and flux density.
As the flux lines in a flux line lattice form a regular arrangement, they are ideal candidates for diffraction experiments. To probe this structure we use neutrons, whose intrinsic magnetic moment weakly interacts with the periodic magnetic potential caused by the presence of vortices. Scattering of the neutrons due the variations in this potential will depend on their spatial proximity to the flux lines. As the spacing of flux lines is typically 100 times that of the cold neutron wavelengths available, the angle of diffraction is very small, typically 1 degree. The small angle of scatter renders the technique the acronym SANS, standing for "small-angle neutron scattering." To allow the diffracted signal to clearly separate from the undiffracted signal, we need large-scale equipment, such as 20m long detectors after the sample and 20m of beam collimation before the sample (which controls the shape and spread of the beam). Of course, we also need a source of neutrons! We in Birmingham use instruments at facilities in mainland Europe for our experiments; most often the reactor source Institut Laue-Langevin (ILL), Grenoble, France, and the spallation source at the Paul Scherrer Institut, north of Zurich in Switzerland. For a slightly more detailed introduction to SANS and some of the group's results in recent years, click here.
Recent Work
The superconducting materials I am most interested in are cuprate and heavy fermion superconductors. As an example of recent work I have been involved in, we have performed recent experiments on the D11 instrument at ILL on the cuprate superconductor Sr0.9La0.1CuO2 (SLCO). SLCO has proved to be of interest due to the fact that work carried out on the compound, using various techniques, suggests that this compound may possess a conventional (s-wave) pairing symmetry, reflecting a conventional pairing mechanism. This is contrary to the belief that all the cuprates possess d-wave pairing symmetry.
We performed some SANS measurements on a polycrystalline sample provided by our Korean collaborators and observed a highly disordered flux line lattice established in the sample. We deduced flux line lattice disorder not only from the absence of clear Bragg diffraction peaks but also from the extremely broad width of the rocking curves. The diffraction pattern at 1.5T on the right shows whilst we did obtain a clear diffraction signal, there are no clear peaks of intensity above Poissonian noise. The signal is distributed about the undiffracted beam, which has been masked in the centre so the diffracted signal from the flux lines is seen. Due to the signal distribution the choice of central mask size was important and was determined by ensuring to obscure the geometrical beamstop in the instrument (in place to stop overloading of the detector) and also the optimisation of the statistics. |
The diffraction image of a summed rocking curve taken over 1.6deg at 1.5T and 2K |
Perhaps the most important result of the experiment was the dependence of the diffracted intensity as a function of temperature. On the right I have fitted the data points at 1.6T with a conventional s-wave BCS model fitting for the gap-size value, and also a prediction for a d-wave model. The data is better represented by the s-wave model, and I deduce a gap size of D(0)=1.70(8)kBTc. This is remarkably close to the weak-coupling limit BCS value of D(0)=1.76kBTc. However, at this field in SLCO, we are not in the true London approximation and various corrections to the observed intensity are required, such as considering the effects of finite vortex core size. By considering such corrections this gap-value increases to D(0)=2.7(1)kBTc. Thus the results not only point to conventional pairing but that this is also indicative of "strong-coupling" behaviour. |
The superfluid density as a function of temperature in an applied field of 1.6T |
What we have concluded is that the data is not well-represented by a d-wave model, and a conventional s-wave pairing model provides a better description. However, the strong-coupling nature of the superconductivity suggests a conventional mechanism may not be an appropriate description. It is not clear why the pairing might be s-wave in this compound though I think it could be derived from the unique crystal structure SLCO possesses and the corresponding influence of this on the Fermi surface evolution with doping. The crystal structure is tetragonal where both a and b are larger than c, and also interestingly x(0)c > c which indicates strong interplanar coupling down to zero temperature, unfamiliar to the High-Tc cuprates. It is possible that the gain in condensation energy is great enough to overcome the increase in energy due to Coulombic repulsion between the the CuO2 planes. There is as yet no theoretical consensus on the superconducting mechanism in SLCO.
For a more detailed discussion and references see J.S.White et al., Journ. Phys.: Cond. Matt. 20, 104237 (2008).